Detected at the two LIGO sites, one in Louisiana and one in Washington State
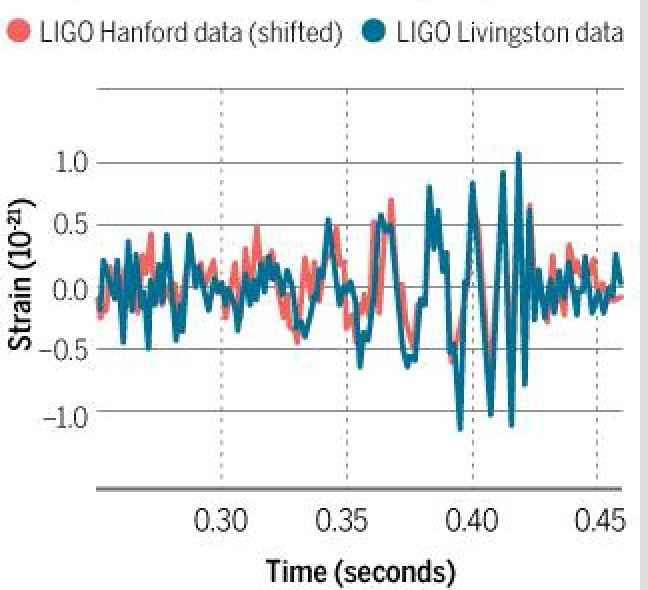
The Laser Interferometer Gravitational-Wave Observatory (LIGO) is a large-scale physics experiment and observatory to detect gravitational waves. LIGO is a joint project between scientists at MIT, Caltech, and many other colleges and universities.




XENON1T/XENONnT
2012-2017 / ∼2017-2022
Another group of scientists
think dark matter is composed of axions — a hypothetical fundamental particle
that we haven't discovered.
Everything that we see around us — planets, stars, nebulas, and so on — only makes up about 15% of the matter in the universe.
The other 85% of matter is missing. Scientists call this invisible stuff "dark matter" because we can't see it and, so far, no one has directly detected it.
We know dark matter exists only because all the matter that we actually can see can't explain the motion of stars and galaxies in the universe. Not even close.
It's become one of the biggest mysteries in physics. There are a lot of differing theories about what dark matter is made of, and how we might actually find it.
From deep underground caverns to laboratories in space, here are five of the coolest dark matter experiments that are attempting to solve this cosmic mystery.

The Cryogenic Dark Matter Search has set more stringent limits on light dark matter.
Direct Dark Matter Detection
The search is more advanced today than we imagined possible," said ATLAS spokesperson Fabiola Gianotti. "We observe in our data clear signs of a new particle, at the level of 5 sigma, in the mass region around 126 GeV. The outstanding performance of the LHC and ATLAS and the huge efforts of many people have brought us to this exciting stage. A little more time is needed to finalize these results, and more data and more study will be needed to determine the new particle’s properties."
The Higgs Boson is an unstable particle, living for only the tiniest fraction of a second before decaying into other particles, so experiments can observe it only by measuring the products of its decay. In the Standard Model, a highly successful physics theory that provides a very accurate description of matter, the Higgs Boson is expected to decay to several distinct combinations of particles, or channels, with the distribution among the channels depending on its mass.
ATLAS concentrated its efforts on two complementary channels: Higgs decays to either two photons or to four leptons. Both of these channels have excellent mass resolution; however, the two-photon channel has a modest signal over a large but measured background, and the four-lepton channel has a smaller signal but a very low background. Both channels show a statistically significant excess at about the same place: a mass of around 126 GeV. A statistical combination of these channels and others puts the significance of the signal at 5 sigma, meaning that only one experiment in three million would see an apparent signal this strong in a universe without a Higgs.
The current results are an update on previous analyses shown at a CERN seminar last December and published at the beginning of this year. The December results, based on 7 TeV proton collision data collected in 2011, limited the mass of the Higgs Boson to two narrow windows in the range between about 117 GeV and 129 GeV. A small excess of events above the expected background was seen by both ATLAS and CMS at around 126 GeV, about the mass of an iodine atom.
The next steps for ATLAS, the LHC and the high-energy physics community are to measure the properties of this particle and compare these measurements with the predicted properties of the Higgs Boson. Already some of these properties match the predictions: the fact that it is seen in the predicted channels and at a mass favoured by other, indirect measurements. In the weeks and months ahead, ATLAS will better measure these properties, enabling a clearer picture to emerge about whether this particle is the Higgs Boson, or the first of a larger family of such particles, or something else entirely.
The 2012 data set comes from proton collisions with an increased centre of mass energy of 8 TeV and includes more data (collected in only three months) than was collected in all of 2011. This rapid accumulation of data was possible thanks to the outstanding efforts of the LHC accelerator group. The data set presented at the seminar comes from approximately one quadrillion (million billion) proton collisions.
The ATLAS detector has performed remarkably well, even under the more difficult beam conditions of 2012, and has, with nearly full efficiency, collected high quality data for this search. Powerful computing provided by the worldwide LHC Computing Grid was essential for the reconstruction and analysis of the data.
The LHC is expected to provide ATLAS with double the data again by the end of the 2012, before the beginning of a long shutdown to upgrade the accelerator. When the machine starts up again toward the end of 2014, it will operate at nearly twice its current energy. The new 2012 data and the data generated by the improved accelerator will allow scientists to address the questions about the Higgs prompted by today’s announcement as well as other questions fundamental to our knowledge of nature.
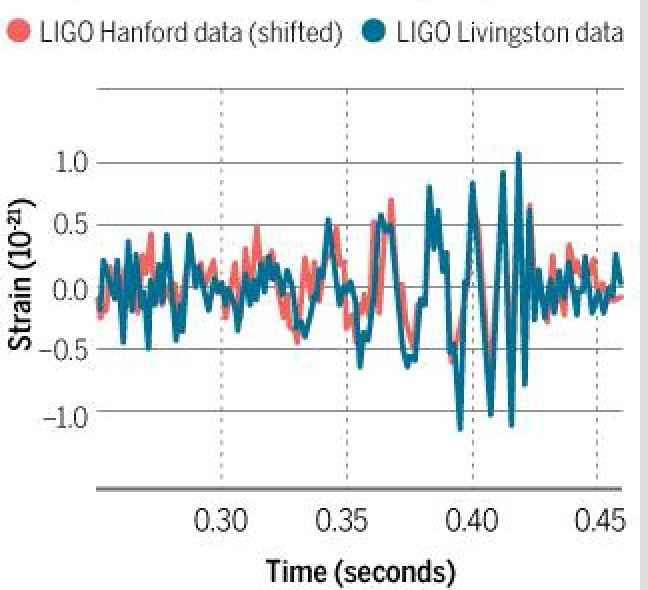
The Laser Interferometer Gravitational-Wave Observatory (LIGO) is a large-scale physics experiment and observatory to detect gravitational waves. LIGO is a joint project between scientists at MIT, Caltech, and many other colleges and universities.



The rotation curve of a disc galaxy (also called a velocity curve) is a plot of the orbital speeds of visible stars or gas in that galaxy versus their radial distance from that galaxy's centre. It is typically rendered graphically as a plot.
Astronomers at the University of Birmingham, Academica Sinica in Taiwan, and the Kavli Institute of Physics and Mathematics of the Universe in Japan, have found new evidence that the mysterious dark matter that pervades our universe behaves as predicted by the ‘cold dark matter’ theory known as ‘CDM’.
Dr Nobuhiro Okabe (Academia Sinica) and Dr Graham Smith (Birmingham), used the Subaru telescope in Hawaii to investigate the nature of dark matter by measuring its density in fifty galaxy clusters, the most massive objects in the Universe.
Team member Professor Masahiro is also excited about the future: ‘Combining lensing observations of many galaxy clusters into a single measurement like this is a very powerful technique. Japanese astronomers are preparing to use Subaru Telescope’s new Hyper Suprime-Cam (HSC) to conduct one of the biggest surveys of galaxies in human history. Our new results are a beautiful confirmation of our plan to use HSC for gravitational lensing studies.’
Distribution of the dark matter
They found that the density of dark matter decreases gently from the center of these cosmic giants out to their diffuse outskirts. The fall in dark matter density from the center to the outskirts agrees very closely with the CDM theory.

Η σκοτεινή ύλη
Η σκοτεινή ύλη είναι μια αόρατη κοσμική «ουσία», η βαρύτητα της οποίας πιστεύεται ότι συγκρατεί τους γαλαξίες και τα αντικείμενα του Σύμπαντος στη θέση τους. Σύμφωνα με τους υπολογισμούς των κοσμολόγων το Σύμπαν αποτελείται από τη συμβατική ύλη (την ορατή ύλη) σε ποσοστό μόλις περίπου 5% ενώ η σκοτεινή ύλη υπολογίζεται ότι αντιστοιχεί σε ποσοστό περίπου 27%, με το υπόλοιπο ποσοστό να αντιστοιχεί στην επίσης μυστηριώδη σκοτεινή ενέργεια. Ως σήμερα έχει καταστεί αδύνατο να εντοπίσουμε τη σκοτεινή ύλη. Το μυστήριο της σκοτεινής ύλης χρονολογείται από τη δεκαετία του 1930, όταν οι αστρονόμοι αντιλήφθηκαν ότι η μάζα και η βαρύτητα των σωμάτων που βλέπουμε στο Σύμπαν δεν είναι αρκετές για να εξηγηθεί η κίνηση των γαλαξιών.
Η σκοτεινή ύλη γίνεται αντιληπτή λόγω της βαρυτικής της επίδρασης στους γαλαξίες, οι επιστήμονες όμως δεν έχουν ιδέα από τι αποτελείται. Γνωρίζουν πάντως ότι δεν εκπέμπει, δεν ανακλά και δεν διαθλά την ακτινοβολία, γι' αυτό και είναι κυριολεκτικά αόρατη. Τα τελευταία χρόνια έχουν αναπτυχθεί και ορισμένες «εξωτικές» θεωρίες για τη σκοτεινή ύλη. Κάποιες από αυτές υποστηρίζουν ότι αποτελεί ένα κβαντικό ελάττωμα που απέμεινε μετά τη γέννηση του Σύμπαντος, ότι διαθέτει μάζα σε άλλες διαστάσεις ή ότι συνιστά μια τροποποιημένη μορφή της βαρύτητας. Οι επιστήμονες αρχίζουν επίσης να υποψιάζονται ότι ο όρος «σκοτεινή» ύλη είναι παραπλανητικός και ότι ίσως θα έπρεπε να αντικατασταθεί με τον όρο «διαφανής» ύλη ή απλώς «άγνωστη» ύλη (materia incognita).
Ποιά είναι τα σενάρια που υπάρχουν για την δομή της σκοτεινής ύλης;
1. Να αποτελείται από συνήθη βαρυονική ύλη (μη-εξωτική)
Δηλαδή αντικείμενα MACHO (Massive Astrophysical Compact Halo Objects). Έρευνες όμως έδειξαν ότι τα MACHO μπορούν να δικαιολογήσουν περίπου το 20% της σκοτεινής βαρυονικής ύλης. Ή νέφη μοριακού υδρογόνου στην εξωτερική περιοχή του γαλαξιακού δίσκου, υπόθεση που ενισχύεται από την ανίχνευση γραμμών CO από αέριο σε απόσταση 12 kpc από το κέντρο του γαλαξία.
2. Να αποτελείται από νετρίνα
2. Να αποτελείται από νετρίνα
Τα νετρίνα με μάζα είναι ένας καλός υποψήφιος για την σκοτεινή ύλη.
3. Να αποτελείται από υπερ-συμμετρικά σωματίδια
Να αποτελείται από εξωτική ύλη (νετραλίνο ή selectron ή φωτίνο), δηλαδή από υπερ-συμμετρικά σωματίδια που θα μπορούσαν να έχουν συνενωθεί σε τεράστιες δομές στην άλω των γαλαξιών. Οι θεωρίες "ρήξης" των Υπερσυμμετρικών Θεωριών υποστηρίζουν την ύπαρξη υπερσυμμετρικών σωματιδίων στην γαλαξιακή άλω, ως αντηχήσεις της Μεγάλης Έκρηξης. Σύμφωνα με αυτές τις θεωρίες τα ελαφρύτερα και σταθερότερα υπερσυμμετρικά σωμάτια που δημιουργήθηκαν στο πρώιμο Σύμπαν, έχουν επιβιώσει μέχρι την εποχή μας και εξαϋλώνονται, παράγοντας πολύ ενεργά αντι-πρωτόνια.
Πηγή: Wikipedia
Το πιο ευαίσθητο
πείραμα αναζήτησης της σκοτεινής ύλης από τα έγκατα της Γης
XENON/Roberto
Corrieri
Ο
νέος ανιχνευτής θα ονομάζεται XENON1T και η «καρδιά» του θα αποτελείται από μία
δεξαμενή 3.300 κιλών με υγρό ξένο, το οποίο θα βρίσκεται σε θερμοκρασία περίπου
-100 βαθμών Κελσίου.
XENON1T/XENONnT
3300 kg / 7000 kg
Projected (2017) / Projected (2022) SI 2 × 10−47 cm2 / SI 3 × 10−48 cm2
Projected (2017) / Projected (2022) SI 2 × 10−47 cm2 / SI 3 × 10−48 cm2
Ένας εξελιγμένος ανιχνευτής είναι στα
σκαριά για το Εθνικό Εργαστήριο Gran Sasso στην Ιταλία, κοντά στην πόλη Λ’ Άκουιλα, ώστε
από το φθινόπωρο το Εργαστήριο να φιλοξενεί το πιο ευαίσθητο πείραμα στον κόσμο
για τον εντοπισμό σωματιδίων σκοτεινής ύλης. Η αλήθεια είναι πως η προσπάθεια
εντοπισμού σωματιδίων σκοτεινής ύλης έχει ξεκινήσει εδώ και μία δεκαετία στις
υπόγειες εγκαταστάσεις του Εργαστηρίου, που βρίσκονται 1.400 μέτρα κάτω από την
οροσειρά Gran Sasso. Ωστόσο, χάρις στον καινούριο ανιχνευτή, το πείραμα θα
αναβαθμισθεί εντυπωσιακά, αποκτώντας 100 φορές μεγαλύτερη διακριτική ικανότητα
από αυτήν που έχει σήμερα.
Το συγκεκριμένο πείραμα στην Ιταλία, όπως
και αρκετές ανάλογες έρευνες που γίνονται σε άλλες χώρες, έχουν στόχο να
διαλευκάνουν το μυστήριο της σκοτεινής ύλης, και πιο συγκεκριμένα το είδος των
σωματιδίων που την απαρτίζουν. Παρόλο που είναι «αόρατη» στα τηλεσκόπια, αφού
δεν απορροφά και δεν εκπέμπει ακτινοβολία, η ύπαρξή της προκύπτει από την
επίδραση που ασκεί σε άστρα και γαλαξίες, τα οποία δεν κινούνται όπως προβλέπει
η θεωρία της βαρύτητας.
Ορισμένοι επιστήμονες πιστεύουν πως αυτό
οφείλεται στο γεγονός ότι είναι ελλιπής η βαρυτική θεωρία. Εντούτοις, η
πλειονότητα των φυσικών υποστηρίζει πως οι παρατηρούμενες ασυμφωνίες οφείλονται
όντως στη σκοτεινή ύλη – η οποία μάλιστα, σύμφωνα με τους υπολογισμούς,
κατακλύζει το σύμπαν σε ποσοστό που αγγίζει το 26,8%.
Όσον αφορά τη φύση αυτής της «εξωτικής»
ύλης, μέχρι σήμερα το μόνο που υπάρχει είναι απλώς θεωρίες, με μία από τις
επικρατέστερες προτάσεις να υποστηρίζει πως το «συστατικό» της σκοτεινής ύλης
είναι τα WIMP (Weakly Interacting Massive Particles). Ωστόσο, μέχρι σήμερα δεν
έχει υπάρξει καμία απολύτως πειραματική ένδειξη που να συνηγορεί είτε υπέρ των
WIMP είτε υπέρ κάποιων άλλων υποθετικών σωματιδίων.
Στην
περίπτωση του Εργαστηρίου Gran Sasso, ο νέος ανιχνευτής θα ονομάζεται XENON1T
και η «καρδιά» του θα αποτελείται από μία δεξαμενή 3.300 κιλών με υγρό ξένο, το
οποίο θα βρίσκεται σε θερμοκρασία περίπου -100 βαθμών Κελσίου. Η τεχνική
ανίχνευσης βασίζεται στο γεγονός ότι, αν η σκοτεινή ύλη όντως υπάρχει, τότε
ορισμένα από τα σωματίδιά της που θα φθάσουν μέσα στη δεξαμενή θα
αλληλεπιδράσουν με άτομα ξένου, προκαλώντας την εκπομπή φωτός και ηλεκτρονίων.
Έτσι, από αυτά τα δύο σήματα, οι επιστήμονες θα μπορούν να προσδιορίσουν το
είδος των σωματιδίων αλλά και τα χαρακτηριστικά τους.
Ο XENON1T δεν θα είναι πιο ευαίσθητος μόνο
τον «προκάτοχό» του – τον ανιχνευτή XENON100 που λειτουργεί στο Εργαστήριο Gran
Sasso από το 2008, με μία δεξαμενή 161 κιλών υγρού ξένου. Επίσης, θα έχει 40
φορές μεγαλύτερη διακριτική ικανότητα από τον ανιχνευτή του LUX (Large
Underground Xenon), το οποίο διεξάγεται σε ένα ορυχείο 1.500 μέτρα κάτω από την
επιφάνεια του εδάφους στη Νότια Ντακότα των ΗΠΑ και από το 2013 κατέχει τον τίτλο του
πιο ευαίσθητου πειράματος στον κόσμο.
Ενδεικτικές είναι οι δηλώσεις στον ιστότοπο
physicsworld.com της Έλενα Έιπριλ, καθηγήτριας αστροφυσικής στο πανεπιστήμιο
Κολούμπια που μέλος της επιστημονικής ομάδας του πειράματος στο Εργαστήριο Gran
Sasso. Σύμφωνα με την Έιπριλ, οι υπάρχοντες ανιχνευτές θα πρέπει να
λειτουργήσουν για αιώνες, ώστε να πετύχουν την ίδια ευαισθησία με τον XENON1T.
Την ίδια στιγμή που η υπερκείμενη οροσειρά Gran Sasso, και τα 1.400 μέτρα
πετρωμάτων που θα βρίσκονται πάνω του, θα εξασφαλίζουν εξαιρετική προστασία από
την ακτινοβολία του περιβάλλοντος και ενδεχόμενες εσφαλμένες μετρήσεις.
Another group of scientists
think dark matter is composed of axions — a hypothetical fundamental particle
that we haven't discovered.

MIT
A proton collides
with a lead nucleus, sending a shower of particles through the CMS detector.
The other 85% of matter is missing. Scientists call this invisible stuff "dark matter" because we can't see it and, so far, no one has directly detected it.
We know dark matter exists only because all the matter that we actually can see can't explain the motion of stars and galaxies in the universe. Not even close.
It's become one of the biggest mysteries in physics. There are a lot of differing theories about what dark matter is made of, and how we might actually find it.
From deep underground caverns to laboratories in space, here are five of the coolest dark matter experiments that are attempting to solve this cosmic mystery.
The Neutrino Research
The Neutrino Research at KEK focuses on the accelerator based long baseline neutrino experiment which provides knowledge on the neutrino mass and mixing.

Summary
The Neutrino Research at KEK focuses on the accelerator based long baseline neutrino experiment which provides knowledge on the neutrino mass and mixing. Currently, the T2K experiment, whose primary aim is to discover muon neutrino to electron neutrino conversion phenomenon, is being conducted. The T2K experiment has announced the discovery of above neutrino conversion phenomenon in 2013.CDMS result covers new ground in search for dark matter
02/28/14The Cryogenic Dark Matter Search has set more stringent limits on light dark matter.
Scientists looking for dark matter face a serious challenge: No one knows what dark matter particles look like. So their search covers a wide range of possible traits—different masses, different probabilities of interacting with regular matter.
Today, scientists on the Cryogenic Dark Matter Search experiment, or CDMS, announced they have shifted the border of this search down to a dark-matter particle mass and rate of interaction that has never been probed.
“We’re pushing CDMS to as low mass as we can,” says Fermilab physicist Dan Bauer, the project manager for CDMS. “We’re proving the particle detector technology here.”
Their result, which does not claim any hints of dark matter particles, contradicts a result announced in January by another dark matter experiment, CoGeNT, which uses particle detectors made of germanium, the same material as used by CDMS.
To search for dark matter, CDMS scientists cool their detectors to very low temperatures in order to detect the very small energies deposited by the collisions of dark matter particles with the germanium. They operate their detectors half of a mile underground in a former iron ore mine in northern Minnesota. The mine provides shielding from cosmic rays that could clutter the detector as it waits for passing dark matter particles.
Today’s result carves out interesting new dark matter territory for masses below 6 billion electronvolts. The dark matter experiment Large Underground Xenon, or LUX, recently ruled out a wide range of masses and interaction rates above that with the announcement of its first result in October 2013.
Scientists have expressed an increasing amount of interest of late in the search for low-mass dark matter particles, with CDMS and three other experiments—DAMA, CoGeNT and CRESST—all finding their data compatible with the existence of dark matter particles between 5 billion and 20 billion electronvolts. But such light dark-matter particles are hard to pin down. The lower the mass of the dark-matter particles, the less energy they leave in detectors, and the more likely it is that background noise will drown out any signals.
Even more confounding is the fact that scientists don’t know whether dark matter particles interact in the same way in detectors built with different materials. In addition to germanium, scientists use argon, xenon, silicon and other materials to search for dark matter in more than a dozen experiments around the world.
“It’s important to look in as many materials as possible to try to understand whether dark matter interacts in this more complicated way,” says Adam Anderson, a graduate student at MIT who worked on the latest CDMS analysis as part of his thesis. “Some materials might have very weak interactions. If you only picked one, you might miss it.”
Scientists around the world seem to be taking that advice, building different types of detectors and constantly improving their methods.
“Progress is extremely fast,” Anderson says. “The sensitivity of these experiments is increasing by an order of magnitude every few years.”
Today, scientists on the Cryogenic Dark Matter Search experiment, or CDMS, announced they have shifted the border of this search down to a dark-matter particle mass and rate of interaction that has never been probed.
“We’re pushing CDMS to as low mass as we can,” says Fermilab physicist Dan Bauer, the project manager for CDMS. “We’re proving the particle detector technology here.”
Their result, which does not claim any hints of dark matter particles, contradicts a result announced in January by another dark matter experiment, CoGeNT, which uses particle detectors made of germanium, the same material as used by CDMS.
To search for dark matter, CDMS scientists cool their detectors to very low temperatures in order to detect the very small energies deposited by the collisions of dark matter particles with the germanium. They operate their detectors half of a mile underground in a former iron ore mine in northern Minnesota. The mine provides shielding from cosmic rays that could clutter the detector as it waits for passing dark matter particles.
Today’s result carves out interesting new dark matter territory for masses below 6 billion electronvolts. The dark matter experiment Large Underground Xenon, or LUX, recently ruled out a wide range of masses and interaction rates above that with the announcement of its first result in October 2013.
Scientists have expressed an increasing amount of interest of late in the search for low-mass dark matter particles, with CDMS and three other experiments—DAMA, CoGeNT and CRESST—all finding their data compatible with the existence of dark matter particles between 5 billion and 20 billion electronvolts. But such light dark-matter particles are hard to pin down. The lower the mass of the dark-matter particles, the less energy they leave in detectors, and the more likely it is that background noise will drown out any signals.
Even more confounding is the fact that scientists don’t know whether dark matter particles interact in the same way in detectors built with different materials. In addition to germanium, scientists use argon, xenon, silicon and other materials to search for dark matter in more than a dozen experiments around the world.
“It’s important to look in as many materials as possible to try to understand whether dark matter interacts in this more complicated way,” says Adam Anderson, a graduate student at MIT who worked on the latest CDMS analysis as part of his thesis. “Some materials might have very weak interactions. If you only picked one, you might miss it.”
Scientists around the world seem to be taking that advice, building different types of detectors and constantly improving their methods.
“Progress is extremely fast,” Anderson says. “The sensitivity of these experiments is increasing by an order of magnitude every few years.”
Direct Dark Matter Detection
A leading hypothesis on the nature of Dark Matter is
that it is comprised of Weakly Interacting Massive Particles, or WIMPs, that
were produced moments after the Big Bang. If WIMPs are the dark matter, then
their presence in our galaxy may be detectable via scattering from atomic
nuclei in detectors as shown in this cartoon:
To shield the experiment from backgrounds from cosmic rays the
detectors are located deep underground - sometimes in active (SNOLAB: 6000
feet) or inactive (Soudan: ~2300 feet) mines. However, even in these deep
underground locations the detectors need to be very sensitive.
Because of the relative motion of Earth through the dark matter
halo we expect an annual modulation of the dark matter signal.
A leading hypothesis on the nature of Dark Matter is that it is comprised of Weakly Interacting Massive Particles, or WIMPs, that were produced moments after the Big Bang. If WIMPs are the dark matter, then their presence in our galaxy may be detectable via scattering from atomic nuclei in detectors as shown in this cartoon:
Range of stop and neutralino masses probed by the search for direct stop pair-production in the single-lepton decay mode (SUS-13-011). The combinations of stop and neutralino masses inside the contours are excluded by the experimental results. The red contour assumes that the stop decays to a top quark and a neutralino, the blue contour assumes that the stop decays to a bottom quark and a chargino.
To σημαντικότερο και ακριβότερο επιστημονικό πείραμα που έχει πραγματοποιηθεί ποτέ στο Διάστημα, το Άλφα Μαγνητικό Φασματόμετρο ή AMS, έχει καταγράψει στον ένα χρόνο λειτουργίας του 18 δισεκατομμύρια προσκρούσεις κοσμικών ακτίνων, ανακοίνωσαν οι υπεύθυνοί του στο CERN.
Εγκατεστημένο στο εξωτερικό του Διεθνούς Διαστημικού Σταθμού, το γιγάντιο όργανο καταγράφει φαινόμενα υψηλότερης ενέργειας από ό,τι ο LHC σε μια προσπάθεια να ανακαλύψει νέες, εξωτικές μορφές ύλης.
O AMS, «παιδί» του νομπελίστα φυσικού Σάμιουελ Τινγκ, μεταφέρθηκε πέρυσι σε τροχιά στη διάρκεια της τελευταίας αποστολής διαστημικού λεωφορείου. Οι αστροναύτες της ιστορικής αποστολής βρέθηκαν την Τετάρτη στο CERN στη Γενεύη για να γιορτάσουν τους 14 μήνες λειτουργίας του. Ο ανιχνευτής «είναι το αποκορύφωμα των επιστημονικών ερευνών που πραγματοποιούνται στον ISS» σχολίασε ο κυβερνήτης της αποστολής Μαρκ Κέλι.
Στην καρδιά του μηχανήματος των 2 δισεκατομμυρίων δολαρίων είναι ένας μαγνήτης, ο οποίος διοχετεύει σε μια σειρά από ανιχνευτές τα σωματίδια που καταφθάνουν από το Διάστημα με ακραίες ταχύτητες. Δεδομένα για τα ηλεκτρικά φορτία, τα επίπεδα ενέργειας και άλλες παραμέτρους των εισερχόμενων σωματιδίων συλλέγονται 25.000 φορές το δευτερόλεπτο και μεταδίδονται στο Κέντρο Ελέγχου της NASA στο Χιούστον, από όπου διαβιβάζονται στο CERN για ανάλυση.
Τα εισερχόμενα σωματίδια έχουν ενέργεια έως και 9 TeV (τρισεκατομμύρια ηλεκτρονιοβόλτ), πολύ υψηλότερη από την ενέργεια των πρωτονίων που συγκρούονται στο Μεγάλο Επιταχυντή Αδρονίων του CERN.
Ο νομπελίστας Σάμιουελ Τινγκ, σήμερα καθηγητής στο ΜΙT, παραδέχτηκε ότι ο σχεδιασμός και η υλοποίηση του πειράματος ήταν πιο δύσκολη από ό,τι είχε φανταστεί: «Δεδομένων των δυσκολιών που συνάντησα, έχω πει στους συνεργάτες μου ότι είναι απίθανο να βρεθούν άνθρωποι αρκετά ανόητοι ώστε να επαναλάβουν αυτό το πείραμα στα επόμενα 40 με 50 χρόνια» δήλωσε.
Ο AMS έχει αναλάβει να μελετήσει τρεις μυστηριώδεις μορφές ύλης που δεν απαντώνται στη Γη, την αντιύλη, τη σκοτεινή ύλη και τα «παραδοξόνια».
To σημαντικότερο και ακριβότερο επιστημονικό πείραμα που έχει πραγματοποιηθεί ποτέ στο Διάστημα, το Άλφα Μαγνητικό Φασματόμετρο ή AMS, έχει καταγράψει στον ένα χρόνο λειτουργίας του 18 δισεκατομμύρια προσκρούσεις κοσμικών ακτίνων, ανακοίνωσαν οι υπεύθυνοί του στο CERN.
Εγκατεστημένο στο εξωτερικό του Διεθνούς Διαστημικού Σταθμού, το γιγάντιο όργανο καταγράφει φαινόμενα υψηλότερης ενέργειας από ό,τι ο LHC σε μια προσπάθεια να ανακαλύψει νέες, εξωτικές μορφές ύλης.
O AMS, «παιδί» του νομπελίστα φυσικού Σάμιουελ Τινγκ, μεταφέρθηκε πέρυσι σε τροχιά στη διάρκεια της τελευταίας αποστολής διαστημικού λεωφορείου. Οι αστροναύτες της ιστορικής αποστολής βρέθηκαν την Τετάρτη στο CERN στη Γενεύη για να γιορτάσουν τους 14 μήνες λειτουργίας του. Ο ανιχνευτής «είναι το αποκορύφωμα των επιστημονικών ερευνών που πραγματοποιούνται στον ISS» σχολίασε ο κυβερνήτης της αποστολής Μαρκ Κέλι.
![]() |
Mark Kelly (C) said AMS was the most significant experiment on the space station |
Στην καρδιά του μηχανήματος των 2 δισεκατομμυρίων δολαρίων είναι ένας μαγνήτης, ο οποίος διοχετεύει σε μια σειρά από ανιχνευτές τα σωματίδια που καταφθάνουν από το Διάστημα με ακραίες ταχύτητες. Δεδομένα για τα ηλεκτρικά φορτία, τα επίπεδα ενέργειας και άλλες παραμέτρους των εισερχόμενων σωματιδίων συλλέγονται 25.000 φορές το δευτερόλεπτο και μεταδίδονται στο Κέντρο Ελέγχου της NASA στο Χιούστον, από όπου διαβιβάζονται στο CERN για ανάλυση.
Τα εισερχόμενα σωματίδια έχουν ενέργεια έως και 9 TeV (τρισεκατομμύρια ηλεκτρονιοβόλτ), πολύ υψηλότερη από την ενέργεια των πρωτονίων που συγκρούονται στο Μεγάλο Επιταχυντή Αδρονίων του CERN.
Ο νομπελίστας Σάμιουελ Τινγκ, σήμερα καθηγητής στο ΜΙT, παραδέχτηκε ότι ο σχεδιασμός και η υλοποίηση του πειράματος ήταν πιο δύσκολη από ό,τι είχε φανταστεί: «Δεδομένων των δυσκολιών που συνάντησα, έχω πει στους συνεργάτες μου ότι είναι απίθανο να βρεθούν άνθρωποι αρκετά ανόητοι ώστε να επαναλάβουν αυτό το πείραμα στα επόμενα 40 με 50 χρόνια» δήλωσε.
Ο AMS έχει αναλάβει να μελετήσει τρεις μυστηριώδεις μορφές ύλης που δεν απαντώνται στη Γη, την αντιύλη, τη σκοτεινή ύλη και τα «παραδοξόνια».
On 4 July, 2012, the ATLAS experiment presented a
preview of its updated results on the search for the Higgs Boson. The results
were shown at a seminar held jointly at CERN and via video link at ICHEP, the
International Conference for High Energy Physics in Melbourne, Australia, where
detailed analyses will be presented later this week. At CERN, preliminary
results were presented to scientists on site and via webcast to their
colleagues located in hundreds of institutions around the world.
The search is more advanced today than we imagined possible," said ATLAS spokesperson Fabiola Gianotti. "We observe in our data clear signs of a new particle, at the level of 5 sigma, in the mass region around 126 GeV. The outstanding performance of the LHC and ATLAS and the huge efforts of many people have brought us to this exciting stage. A little more time is needed to finalize these results, and more data and more study will be needed to determine the new particle’s properties."
The Higgs Boson is an unstable particle, living for only the tiniest fraction of a second before decaying into other particles, so experiments can observe it only by measuring the products of its decay. In the Standard Model, a highly successful physics theory that provides a very accurate description of matter, the Higgs Boson is expected to decay to several distinct combinations of particles, or channels, with the distribution among the channels depending on its mass.
ATLAS concentrated its efforts on two complementary channels: Higgs decays to either two photons or to four leptons. Both of these channels have excellent mass resolution; however, the two-photon channel has a modest signal over a large but measured background, and the four-lepton channel has a smaller signal but a very low background. Both channels show a statistically significant excess at about the same place: a mass of around 126 GeV. A statistical combination of these channels and others puts the significance of the signal at 5 sigma, meaning that only one experiment in three million would see an apparent signal this strong in a universe without a Higgs.
The current results are an update on previous analyses shown at a CERN seminar last December and published at the beginning of this year. The December results, based on 7 TeV proton collision data collected in 2011, limited the mass of the Higgs Boson to two narrow windows in the range between about 117 GeV and 129 GeV. A small excess of events above the expected background was seen by both ATLAS and CMS at around 126 GeV, about the mass of an iodine atom.
The next steps for ATLAS, the LHC and the high-energy physics community are to measure the properties of this particle and compare these measurements with the predicted properties of the Higgs Boson. Already some of these properties match the predictions: the fact that it is seen in the predicted channels and at a mass favoured by other, indirect measurements. In the weeks and months ahead, ATLAS will better measure these properties, enabling a clearer picture to emerge about whether this particle is the Higgs Boson, or the first of a larger family of such particles, or something else entirely.
The 2012 data set comes from proton collisions with an increased centre of mass energy of 8 TeV and includes more data (collected in only three months) than was collected in all of 2011. This rapid accumulation of data was possible thanks to the outstanding efforts of the LHC accelerator group. The data set presented at the seminar comes from approximately one quadrillion (million billion) proton collisions.
The ATLAS detector has performed remarkably well, even under the more difficult beam conditions of 2012, and has, with nearly full efficiency, collected high quality data for this search. Powerful computing provided by the worldwide LHC Computing Grid was essential for the reconstruction and analysis of the data.
The LHC is expected to provide ATLAS with double the data again by the end of the 2012, before the beginning of a long shutdown to upgrade the accelerator. When the machine starts up again toward the end of 2014, it will operate at nearly twice its current energy. The new 2012 data and the data generated by the improved accelerator will allow scientists to address the questions about the Higgs prompted by today’s announcement as well as other questions fundamental to our knowledge of nature.
Mass distribution for the two-photon channel. The
strongest evidence for this new particle comes from analysis of events
containing two photons. The smooth dotted line traces the measured background
from known processes. The solid line traces a statistical fit to the signal
plus background. The new particle appears as the excess around 126.5 GeV. The
full analysis concludes that the probability of such a peak is three chances in
a million.
Mass distribution for the four-lepton channel. The
search with the purest expected signal is done by examining events with two Z
bosons that have decayed to pairs of electrons or muons. In the region from 120
to 130 GeV, 13 events are seen where only 5.3 were expected. The complete
analysis concludes that the probability of such an excess would be three times
in ten thousand if there were no new particle
Experimental limits from ATLAS on Standard Model
Higgs production in the mass range 110-600 GeV. The solid curve reflects the
observed experimental limits for the production of a Higgs of each possible
mass value (horizontal axis). The region for which the solid curve dips below
the horizontal line at the value of 1 is excluded with a 95% confidence level
(CL). The dashed curve shows the expected limit in the absence of the Higgs
boson, based on simulations. The green and yellow bands correspond
(respectively) to 68%, and 95% confidence level regions from the expected
limits. Higgs masses in the narrow range 123-130 GeV are the only masses not
excluded at 95% CL.
The probability of background to produce a
signal-like excess, for all the Higgs boson masses tested. At almost all
masses, the probability (solid curve) is at least a few percent; however, at
126.5 GeV it dips to 3x10-7, or one chance in three million, the '5-sigma'
gold-standard normally used for the discovery of a new particle. A Standard
Model Higgs boson with that mass would produce a dip to 4.6 sigma.
Δεν υπάρχουν σχόλια:
Δημοσίευση σχολίου